If dark matter and antimatter collided, you'd witness an incredible energy release, far surpassing that of nuclear reactions. This phenomenon could fundamentally alter our understanding of the universe. While dark matter might ignore antimatter, potential interactions could reveal new particles and hidden dimensions. Additionally, such collisions could generate gamma rays and neutrinos, reshaping cosmic structures like stars and galaxies. The challenges of detection and experimentation are immense, but the implications are staggering. There's still much to uncover about how these cosmic elements interact, and what it could mean for the fabric of reality.
Essential Insights
- Dark matter's interactions with antimatter are largely speculative, with current theories suggesting minimal or collisionless interactions due to dark matter's elusive nature.
- Matter-antimatter collisions yield immense energy through annihilation, potentially producing gamma rays and neutrinos, but dark matter's properties complicate this process.
- Theoretical models propose that dark matter might ignore antimatter, leading to unique cosmic implications and potential new physics insights about the universe.
- Collisions between antimatter and dark matter candidates could reveal hidden dimensions or exotic particles, reshaping our understanding of cosmological theories.
- Advanced experiments are needed to investigate dark matter-antimatter interactions, which may uncover fundamental properties and address the matter-antimatter imbalance in the universe.
Understanding Dark Matter and Antimatter
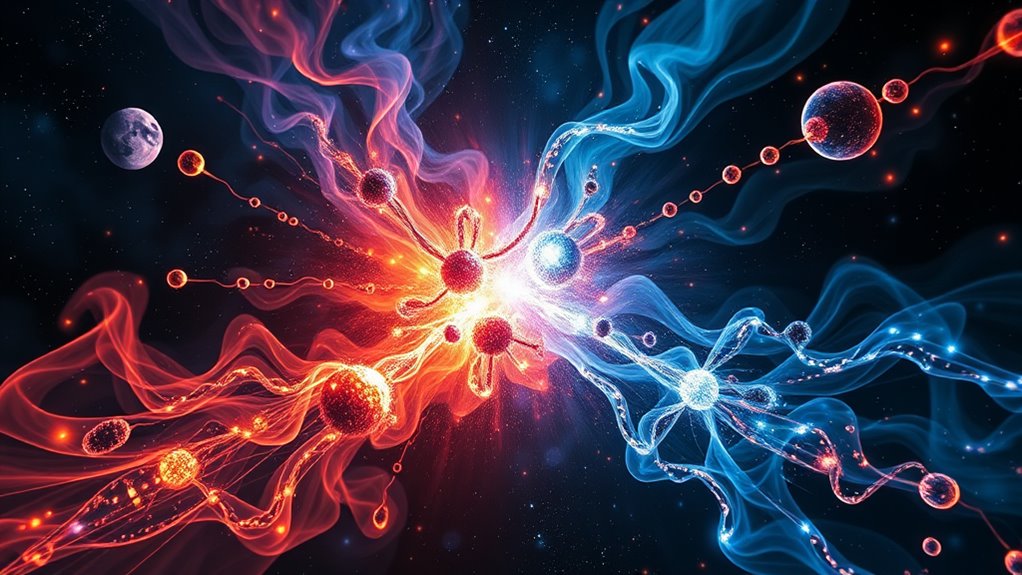
When you plunge into the domains of dark matter and antimatter, you'll find two captivating yet elusive components of our universe.
Dark matter, constituting about 26.8% of the universe's mass-energy content, originates from gravitational effects that defy explanation by general relativity alone. It's a hypothetical form of matter that doesn't interact with light or electromagnetic radiation, making its detection a significant challenge. The most accepted theories suggest that dark matter consists of undiscovered subatomic particles, such as WIMPs or axions, or even primordial black holes. Its presence is vital for understanding the structure and formation of galaxies, as it accounts for approximately 85% of total mass in the universe.
On the flip side, antimatter is composed of antiparticles, which have the same mass as ordinary matter but opposite electric charges. You might encounter positrons and antiprotons as examples of antimatter particles. Curiously, while antimatter has properties that mirror those of ordinary matter, it behaves the same way under gravity. Despite its intriguing nature, no substantial amounts of antimatter have been assembled due to the complexities involved in its production and handling.
Both dark matter and antimatter play significant roles in the cosmos, influencing everything from galaxy formation to the behavior of particles in cosmic rays.
As you explore these topics, consider how their unique characteristics might interact, especially when you think about the possibilities surrounding their collision. Understanding dark matter origins alongside antimatter properties opens doors to questions that challenge our grasp of the universe.
Interaction Mechanisms Between the Two
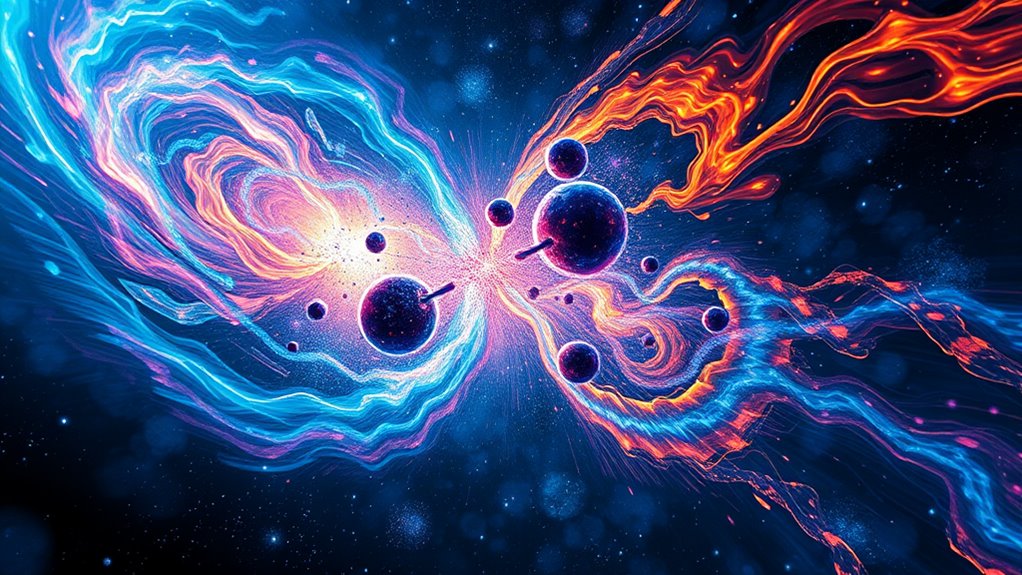
The interaction mechanisms between dark matter and antimatter present a fascinating frontier in modern physics. Researchers have proposed various dark matter theories to explain how these two elusive components of the universe might interact. For instance, the BASE collaboration conducted the first laboratory search for interactions between antimatter and potential dark matter candidates like axions. Using a Penning trap to magnetically hold a single antiproton, scientists measured its spin precession frequency, seeking fluctuations that could suggest interactions with dark matter.
Here's a snapshot of key findings:
Experiment | Observation | Implications |
---|---|---|
BASE Collaboration | No observed difference | Sets an upper limit for potential interactions |
Penning Trap Experiment | Constant spin precession | Indicates no detectable axion effect |
WIMP Models | Weak interactions | Difficulty in detection |
Cosmic Ray Observations | Antihelium detection | Clues about WIMPs and dark matter |
Dark Antimatter Hypothesis | Theoretical exploration | Speculative energy releases |
Despite the lack of observed interactions, theories suggest that dark matter might be "collisionless," meaning it could ignore antimatter altogether. Concepts like dark antimatter and hidden dimensions propose new avenues for understanding the universe. Observations suggest a significant amount of unseen matter exists in the universe, while the elusive nature of dark matter hampers detection, advancements in technology may eventually help us unravel these mysteries, potentially revolutionizing our grasp of antimatter properties and the fundamental forces shaping our reality.
Energy Release From Collisions
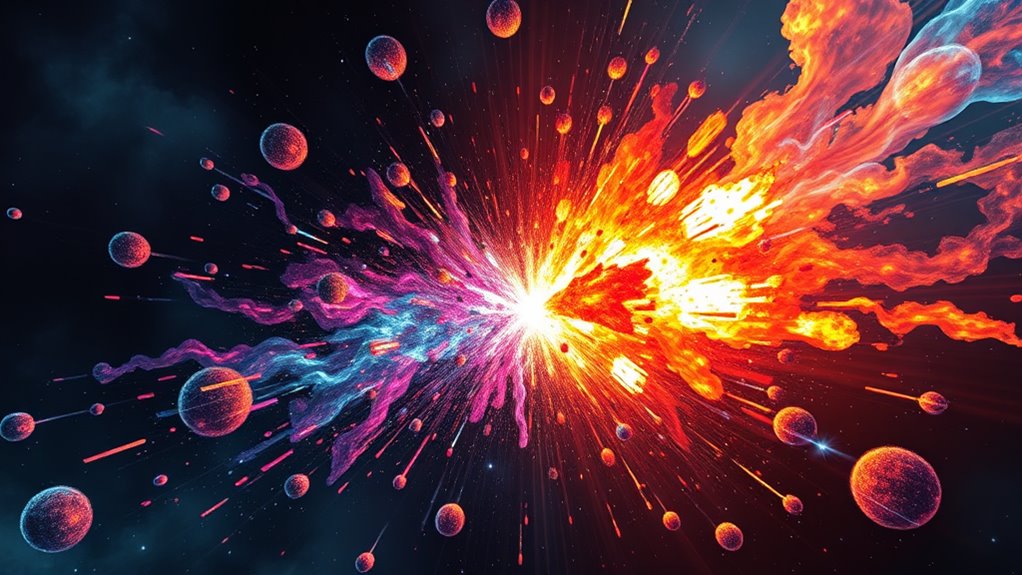
Collisions between matter and antimatter release a staggering amount of energy, making them one of the most potent energy sources known. When these particles collide, they annihilate each other, converting their mass into energy according to Einstein's equation, E=mc². This energy release is a result of intricate energy dynamics, where the annihilation produces gamma rays, neutrinos, and various quark flavors, depending on the particles involved.
For instance, when electrons meet positrons, they yield high-energy gamma rays. In contrast, proton-antiproton collisions can lead to more complex particle transformations, yielding quarks and antiquarks along with heat and light. Even a mere milligram of antimatter can release energy equivalent to a small nuclear bomb, demonstrating the immense scale of energy release. This is particularly significant because antimatter is scarce in the universe, emphasizing the rarity and potential of harnessing such energy sources.
The energy density from these annihilations is unparalleled, far surpassing both chemical and conventional nuclear reactions. You could power a large city for an entire year with just a small amount of antimatter. However, harnessing this energy poses significant challenges due to the volatile nature of the reaction. Advanced laboratory setups are essential for capturing and measuring this energy before the antimatter collides with regular matter.
To accurately measure the energy released, scientists use highly sensitive detectors and magnetic traps to isolate antimatter. Understanding this energy release not only reveals the fundamental properties of antimatter but also sheds light on its interactions, paving the way for potential advancements in energy generation and particle physics.
Theoretical Scenarios and Implications
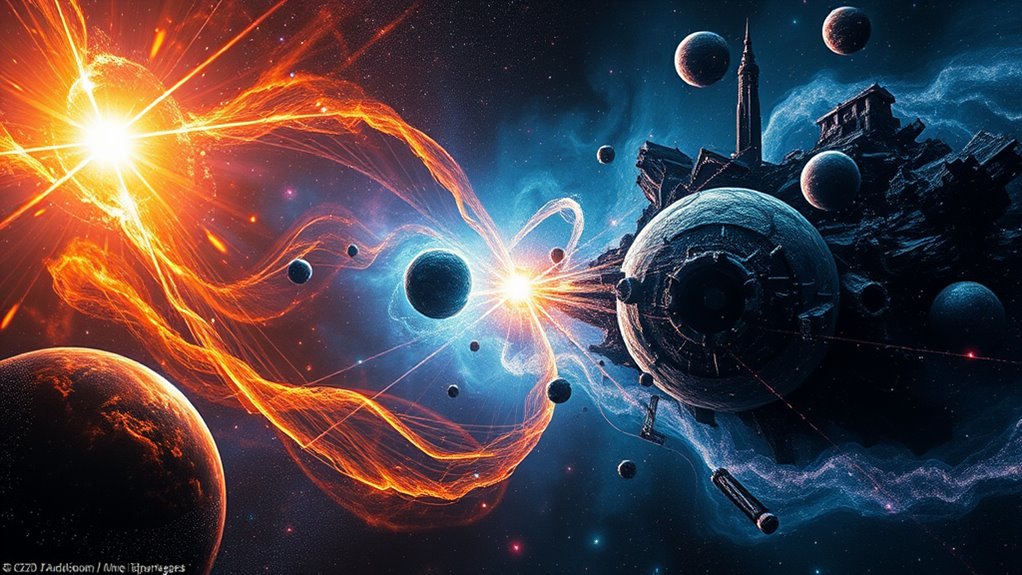
Dark matter and antimatter interactions present intriguing theoretical scenarios that could reshape our understanding of the universe. One possibility is that dark matter, potentially made of weakly interacting massive particles (WIMPs), might completely ignore antimatter. Since WIMPs don't interact much with other forms of matter, including antimatter, you might find that collisions between them yield no observable effects. This collisionless nature means dark matter could pass through antimatter without any destructive reactions.
On the other hand, if interactions do occur, they could lead to groundbreaking revelations. For instance, such collisions could reveal portals to hidden dimensions, releasing exotic particles or energy sources that might power cosmic phenomena. The gravitational waves produced from these events could be invisible, posing significant detection challenges. Additionally, the potential for dark matter to constitute approximately 85% of the universe's total matter highlights its significance in these interactions.
You might also consider the implications of a "dark sector," where new particles emerge from dark matter and antimatter interactions. These particles may influence gravitational fields or contribute to the structure of galaxies in ways we've yet to understand.
Additionally, if dark matter gradually transformed into dark antimatter over eons, it could destabilize the universe's very fabric, leading to catastrophic events like the "Big Rip."
Ultimately, exploring these dark matter theories alongside antimatter properties could illuminate the long-standing mysteries regarding the universe's matter-antimatter imbalance, potentially offering profound insights into its composition and fate. Understanding these interactions could redefine your perception of the cosmos.
Experimental Challenges in Research
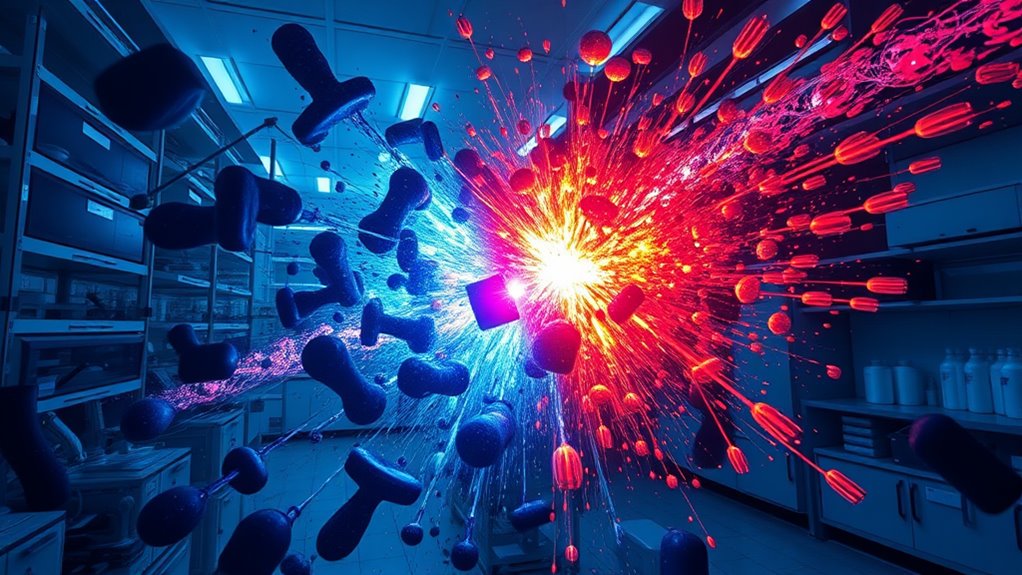
Exploring the interactions between dark matter and antimatter leads to significant experimental challenges that researchers must manage. One of the primary hurdles is the detection of rare interactions, which requires advanced detection techniques to identify elusive particles. To tackle this, specialized devices like Penning traps are utilized to magnetically trap antiprotons and prevent their annihilation with ordinary matter, ensuring that researchers can study them effectively.
The experimental design must also accommodate high-energy collisions, as seen in LHCb experiments where proton-proton and proton-helium collisions occur at energy scales up to 13 TeV. These setups are essential for examining antiproton production and understanding potential interactions with dark matter. Precise magnetic fields are employed to measure properties like the spin precession frequency of antiprotons, which helps set upper limits on dark matter-antimatter interactions. Additionally, the LHCb collaboration's findings highlight the significance of antiprotons from antihyperon decays in total production rates.
Moreover, the collection of collision data presents another layer of complexity. Researchers analyze extensive datasets, such as millions of proton-helium collisions, to refine model predictions and improve understanding of particle behavior. Advanced detectors play an important role in this process, enabling particle identification and measurement with high precision.
The collaborative nature of this research, involving teams from various institutions, further complicates the experimental design. Researchers must work together to share insights and enhance detection techniques.
Cosmic Implications for the Universe
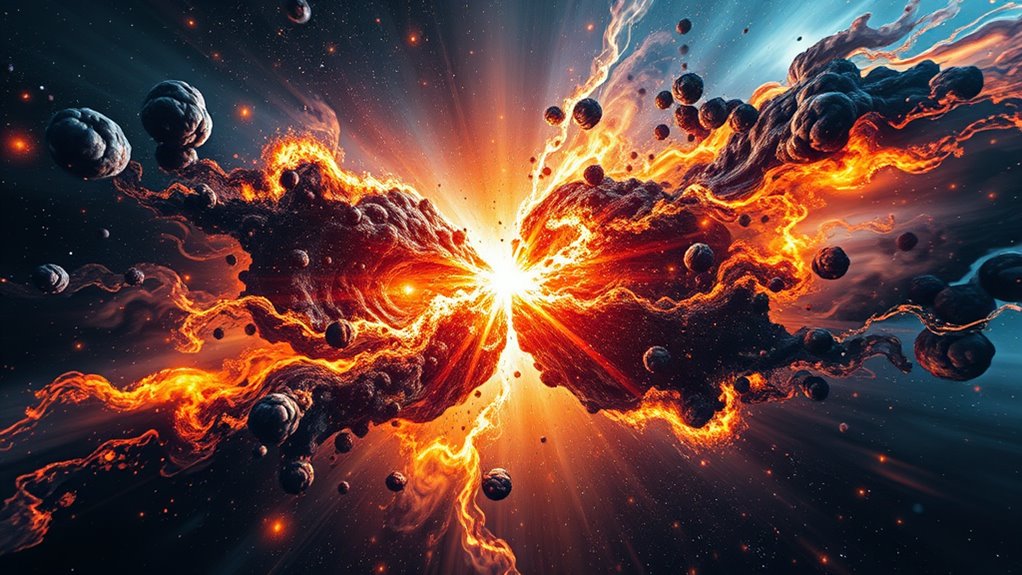
The interactions between dark matter and antimatter could have profound cosmic implications for the universe. When these two enigmatic entities collide, the outcomes might reshape our understanding of cosmic structure and energy. Here are some potential implications to ponder:
- Gateway to New Dimensions: Collisions may reveal portals to alternate dimensions, releasing exotic particles and energy sources.
- Silent Annihilation: Interactions might produce only invisible gravitational waves, leaving no detectable light or heat.
- Cosmic Engine: These collisions could serve as a cosmic engine, potentially powering stars and galaxies through hidden energy mechanisms.
- Dark Sector Particles: The interactions could create particles from a "dark sector," subtly influencing the fabric of space.
- Matter-Antimatter Imbalance: Dark matter interactions might help explain the universe's existing imbalance between matter and antimatter, potentially addressing the violation of matter/anti-matter symmetry.
Understanding these cosmic implications could open doors to discovering alternative physics, as interactions might reveal new particles and energy sources previously unimagined.
The potential for such transformations could also lead to significant shifts in our universe's mass distribution, influencing everything from galactic stability to phenomena like the 'Big Rip.'
With dark matter constituting about 85% of the universe's mass, its interactions with antimatter could fundamentally alter our grasp of gravitational waves and the underlying structure of the cosmos.
As we explore deeper into these interactions, we may uncover a universe rich with complexities waiting to be examined.
Potential Applications of Interactions
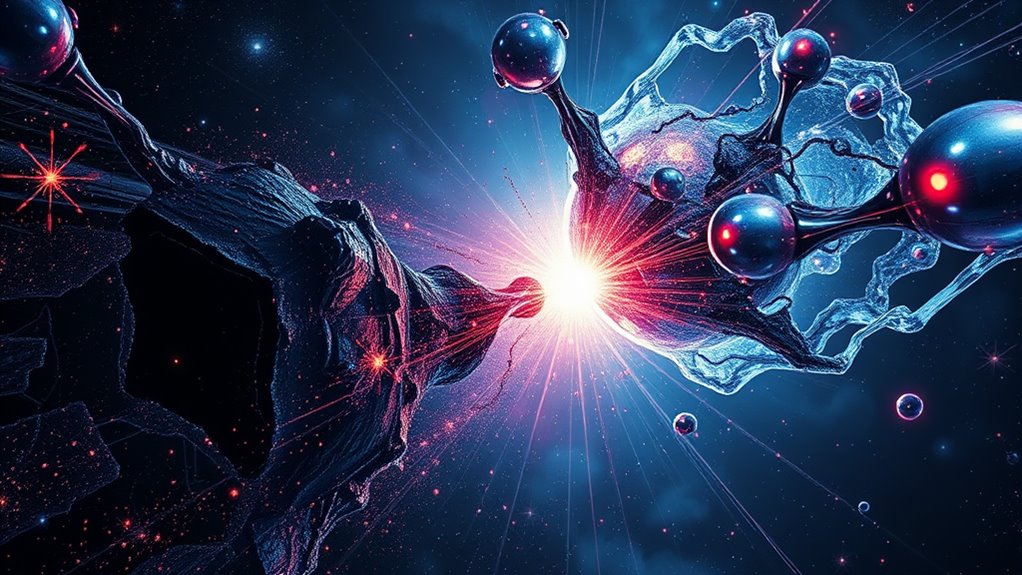
As researchers explore the potential applications of dark matter and antimatter interactions, they uncover groundbreaking possibilities that could transform energy production and technology. The prospect of harnessing energy from these collisions offers a tantalizing glimpse into endless power sources, but with great potential comes significant risks.
To understand these applications better, consider the following table illustrating key aspects:
Aspect | Description |
---|---|
Energy Release | Collisions could release energy comparable to nuclear reactions. |
Technological Challenges | Advanced tech is needed to stabilize volatile reactions. |
Safety Protocols | Strict protocols are essential to prevent catastrophic failures. |
Imagine the possibilities if we could successfully manage these volatile interactions. Energy harvesting from dark matter-antimatter collisions might not only power cities but also propel spaceships across galaxies. However, the technological challenges are formidable. Producing and stabilizing antimatter safely requires innovations and international collaboration, such as the BASE collaboration, which is essential for advancements in this field.
Implementing safety protocols is critical, as any malfunction could lead to uncontrollable energy releases. Researchers are keenly aware of the need for improved detection methods and advanced experiments to monitor these interactions. As the scientific community continues to explore these avenues, the dream of tapping into dark matter and antimatter energy could one day become a reality, revolutionizing our understanding of energy production and technology.
Frequently Asked Questions
What Are the Current Theories About Dark Matter's Composition?
Current theories about dark matter's composition highlight various dark matter candidates, each explaining particle interactions differently.
For instance, WIMPs and axions propose particles with unique properties, while self-interacting dark matter suggests that dark matter can interact among itself.
Modified Newtonian Dynamics offers an alternative perspective, adjusting classical laws to account for observed phenomena.
Each theory aims to unravel the mysteries of dark matter, contributing to our understanding of the universe's structure and behavior.
How Does Dark Matter Influence Galaxy Formation and Evolution?
Imagine gazing at the night sky, wondering how galaxies form and evolve.
Dark matter's role is essential; it shapes cosmic structure and facilitates galaxy clustering. You see, dark matter creates gravitational wells that pull ordinary matter together, allowing galaxies to form.
Its presence influences everything from star formation rates to the distribution of stars within these galaxies. Without dark matter, the universe's grand design would be vastly different, leaving our night sky eerily empty.
Are There Known Examples of Dark Matter Effects in the Universe?
Yes, there are several known examples of dark matter effects in the universe.
One prominent example is gravitational lensing, where dark matter's presence distorts and magnifies light from distant galaxies.
This phenomenon allows you to observe galaxy clusters and map the distribution of dark matter, revealing how it influences the structure of the cosmos.
What Are the Main Types of Antimatter Studied in Research?
Think of antimatter as the universe's shadow, a mirror reflecting the ordinary matter we understand.
In research, you'll encounter several key types: positrons, antiprotons, and antineutrons, each essential for antimatter production.
You'll also discover exotic antinuclei like antihydrogen and antihelium.
Beyond their fascinating nature, these particles have practical antimatter applications, from medical imaging in PET scans to potential cancer therapies, making them invaluable for both science and health advancements.
How Do Scientists Detect Dark Matter Indirectly?
Scientists detect dark matter indirectly by observing its gravitational effects, like gravitational lensing, which reveals how mass bends light from distant objects.
They also study the cosmic microwave background to spot anomalies linked to dark matter's presence.
By analyzing gamma rays, neutrinos, and cosmic rays, they identify excess particles that hint at dark matter interactions.