If you fell into a black hole, you'd first experience gravitational redshift, where the light you emit appears dimmer and redder to distant observers. As you approach the event horizon, everything seems normal, but time for you would slow immensely compared to those far away. Crossing the horizon means there's no turning back. The intense tidal forces would stretch you dramatically, a process known as spaghettification. Finally, near the singularity, the laws of physics break down, crushing everything to infinite density. Curious about what happens next and how it all ties back to our universe?
Essential Insights
- Crossing the event horizon feels smooth, but you become unable to escape the black hole's gravitational pull.
- Time appears to slow down for you compared to distant observers due to extreme time dilation effects.
- Tidal forces stretch and compress objects, leading to spaghettification, especially in smaller black holes.
- Once inside, light cannot escape, causing you to become invisible to outside observers.
- You may eventually encounter the singularity, where the laws of physics break down and matter compresses infinitely.
Approaching the Event Horizon
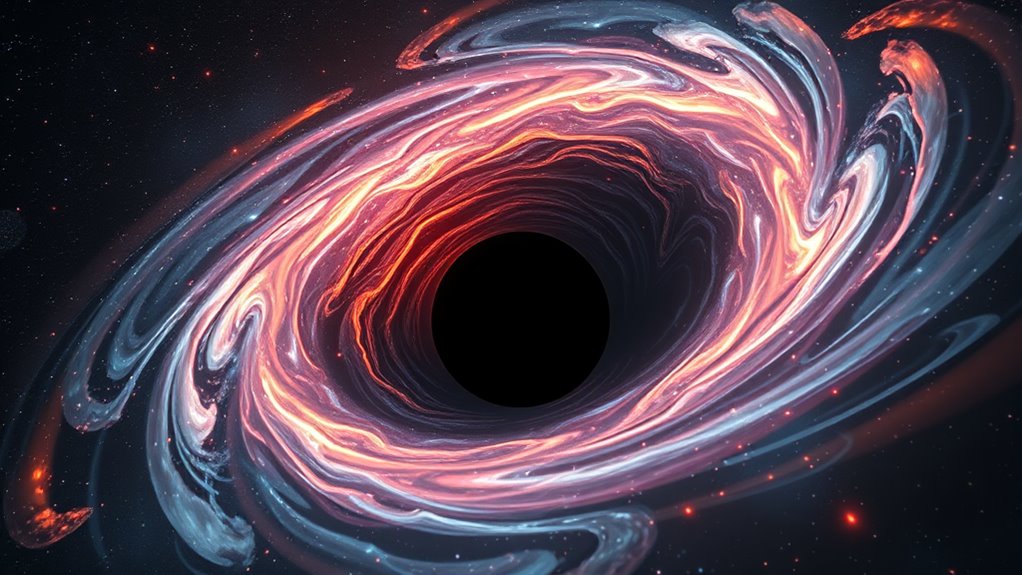
What happens as you approach a black hole's event horizon? You might notice that your surroundings begin to change dramatically. As you get closer, the light from distant stars starts to stretch, causing a phenomenon known as gravitational redshift.
To outside observers, you appear redder and dimmer, making it seem like you're slowing down. In fact, you'll never be seen crossing the event horizon due to the observational limits of light cones; the moment you cross isn't visible to anyone outside.
As you near the horizon, tidal forces become increasingly intense. If you were to stretch out your limbs, you'd experience spaghettification, where the gravitational pull tears you apart long before you actually reach the event horizon. This is akin to how Wilfred Arthur's military career showcased the extreme pressures faced during aerial combat.
This extreme gravity disrupts your material structure, making it impossible to remain intact. You'd also feel an unbounded increase in proper acceleration, meaning you'd struggle to stay stationary near the horizon.
Importantly, the event horizon isn't a physical barrier; it's a mathematical boundary marking the point of no return. There's no special sensation when you cross it, and there's no physical surface to touch.
Instead, it's a finite distance that you can't escape once crossed. All the light and radiation generated inside the event horizon can't escape, sealing your fate, while any light trying to reach outside observers gets stretched and dimmed, rendering your crossing a hidden event.
Time Dilation Effects
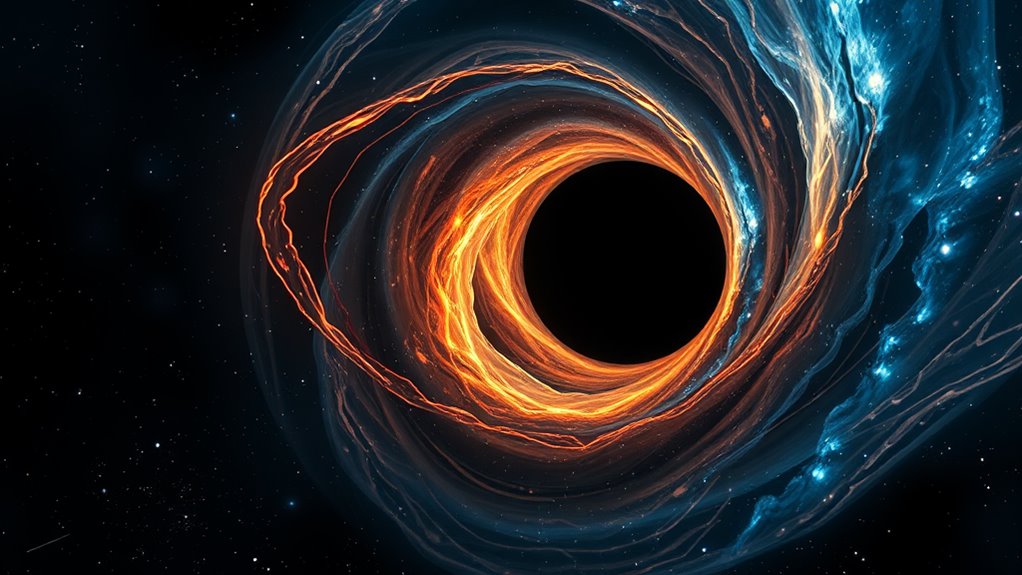
As you approach a black hole, time dilation effects become increasingly pronounced due to the intense gravitational field. According to Einstein's theory of general relativity, this gravitational force curves spacetime, causing a significant alteration in time perception. The closer you get to the black hole, the more pronounced these relativistic effects become.
Using the Schwarzschild metric, we can describe how the basis vectors in the time direction shorten as you move closer to the black hole. Fundamentally, this compression causes intervals of time to pass more slowly for you compared to someone far away. While you won't notice any difference in your own experience of time, distant observers will see your clock ticking slower and slower as you near the event horizon. This phenomenon is especially evident as gravitational time dilation increases with proximity to the black hole.
For them, it seems like you're freezing in time, especially right at that critical boundary. In contrast, you age normally; the time you experience is consistent for you, even if it diverges dramatically from what others perceive.
Once you cross the event horizon, no signals can escape, making it impossible for anyone to witness what happens next.
These effects highlight the unique laboratory that black holes provide for studying time and gravity. Time dilation isn't limited to black holes; it occurs in weaker gravitational fields, too, but the extreme conditions near a black hole amplify these relativistic effects to a staggering degree, reshaping your understanding of time itself.
Gravitational Redshift Explained
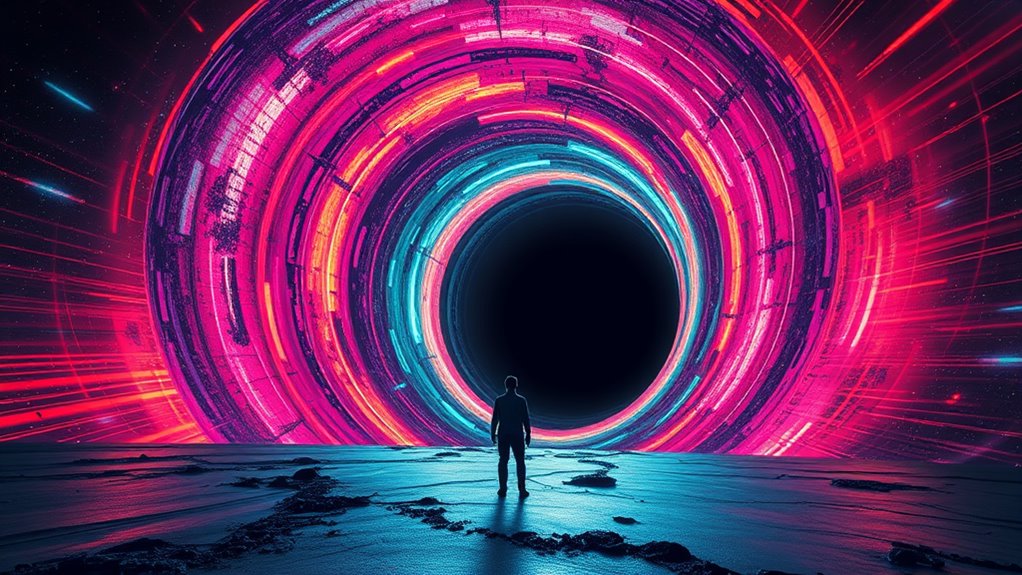
The effects of gravitational redshift become particularly pronounced near a black hole, where light struggles to escape the intense gravitational field. According to Einstein's general theory of relativity, as photons climb out of the black hole's gravitational well, they lose energy and increase in wavelength, resulting in a noticeable redshift. This phenomenon is observable in the light emitted by stars like S0-2, which orbits the supermassive black hole at the center of the Milky Way. During its closest approach, the redshift effect peaks due to the immense gravitational pull. Observations of star S2, which orbits the supermassive black hole, have provided strong evidence supporting general relativity.
Gravitational lensing plays a vital role here, bending the paths of light around massive objects, further complicating photon behavior in these extreme environments. The stronger the gravitational field, the more evident the redshift becomes. Near a black hole's event horizon, this effect can stretch the wavelengths of light beyond the visible spectrum, leaving it entirely redshifted.
From your perspective, if you fell into a black hole, you'd notice that the incoming light appears blueshifted due to the gravitational field's intensity. However, this blueshift is a coordinate-dependent phenomenon, meaning it doesn't affect the local spacetime greatly if the black hole's mass is substantial.
While the intense energy of blueshifted light poses theoretical risks, large black holes mitigate these local impacts, allowing the gravitational redshift to serve as a significant test for general relativity in extreme conditions.
Tidal Forces and Spaghettification
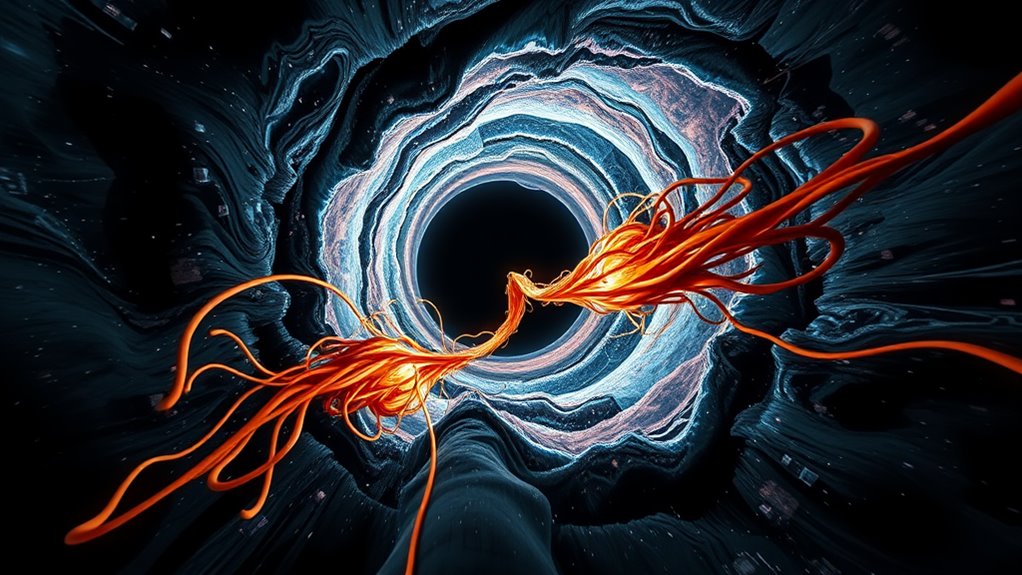
Approaching a black hole, you'll encounter extreme tidal forces that dramatically alter the fabric of any object caught within their grasp. These forces arise from the gravitational gradient, which becomes increasingly steep as you get closer. The difference in gravitational pull between the parts of your body causes tidal stretching, leading to a phenomenon known as spaghettification. Furthermore, as you approach the black hole, the gravitational redshift causes the light emitted from your body to appear increasingly redder to distant observers.
As you near the black hole, the tidal forces start mild but quickly escalate, stretching you in the direction of the black hole while compressing you sideways. Eventually, these forces exceed your elastic limits, causing you to snap apart at your weakest points. You'd be reduced to a string of disconnected atoms, spiraling toward the singularity.
Here's a quick overview of how tidal forces affect objects of different sizes:
Black Hole Size | Effect on Objects |
---|---|
Small Black Holes | Strong tidal forces, spaghettification occurs early. |
Supermassive Black Holes | Weaker tidal forces, less severe deformation until very close to the horizon. |
General Objects | Smaller objects are more susceptible to spaghettification due to their size relative to tidal effects. |
The tidal forces can also disrupt rigid bodies like planets, demonstrating their immense strength. For larger black holes, the spaghettification effect is less pronounced until you approach the event horizon, making the experience somewhat less dramatic compared to smaller black holes. However, the fundamental nature of tidal forces remains the same, leading to an inevitable fate.
Crossing the Event Horizon
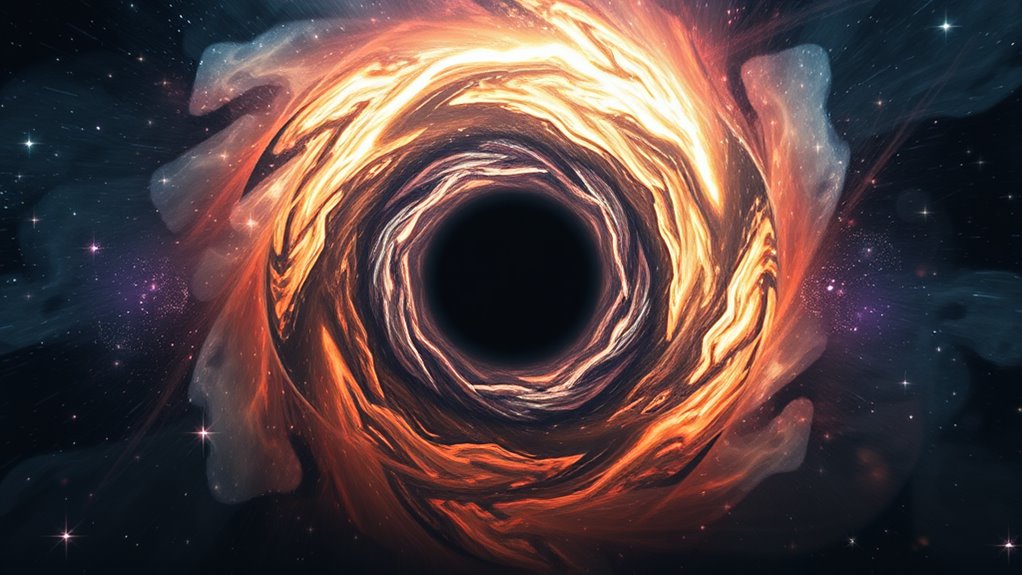
Once you've been subjected to the extreme tidal forces near a black hole, you'll inevitably reach the event horizon, the defining boundary of this cosmic phenomenon. At this point, you've crossed into a domain where escape is impossible. The event horizon implications are profound, marking the point of no return. Though you won't notice an immediate change, your fate is sealed.
Imagine this scenario:
- The cosmos stretches out before you, a swirling tapestry of stars and light.
- Time appears to slow down, creating a surreal sense of detachment.
- The gravitational pull is palpable, tugging at your very essence.
- Light from the universe outside is distorted, bending around the black hole.
From your perspective, crossing the event horizon is a smooth shift, devoid of dramatic effects. However, if an external observer were watching, they'd witness you slowing down, never quite reaching the boundary, thanks to gravitational redshift.
Inside, you can still observe the light from the universe, but signals can no longer escape. This experience is compounded by the fact that smaller black holes exhibit more extreme tidal forces than larger ones, making your journey into the unknown even more intense.
This unique vantage point raises fascinating black hole mysteries. Space-time itself warps around you, creating an experience unlike anything else in the universe.
Despite the calm appearance, you're now enveloped in a zone where the rules of reality bend. The event horizon isn't just a boundary; it's a threshold into the unknown, prompting questions about time, light, and the fate of information that crosses it.
Fate Inside the Black Hole
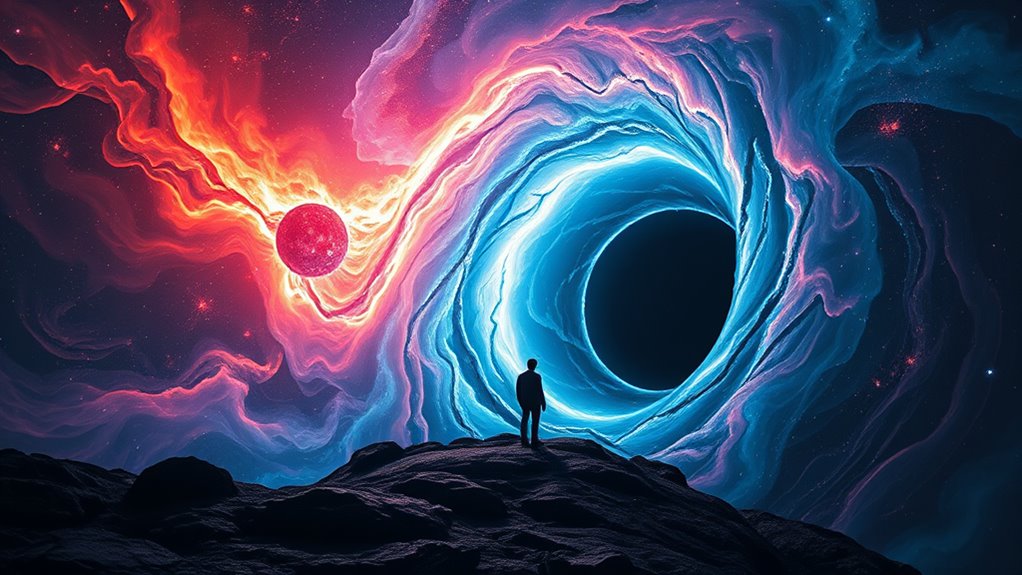
As you drift deeper into the black hole, reality begins to unravel in ways that challenge your understanding of physics. The gravitational forces intensify, leading to extreme tidal effects known as spaghettification. You'll feel your body stretching, pulled apart by the immense gravitational gradient. If you're near a stellar-mass black hole, this occurs even before you reach the event horizon.
Larger black holes offer a brief reprieve, but as you approach the singularity, the forces become catastrophic. Notably, Hawking radiation suggests that black holes may emit energy, leading to an eventual decay and raising questions about their permanence.
Time behaves strangely here. From your perspective, everything seems normal, but for observers far away, time appears to stand still. This phenomenon, known as time dilation, reveals just one of the black hole mysteries that scientists grapple with.
As you continue your descent, you witness gravitational lensing, where the surrounding stars and galaxies warp around you, distorting your view of the cosmos.
Ultimately, however, your journey leads to the singularity, a point of infinite density where all paths converge. At this stage, atoms break apart into fundamental particles, and the very fabric of your existence faces obliteration.
The cosmic implications of this encounter are profound. Once you cross the event horizon, all information about your matter seems lost to the universe, raising the infamous black hole information paradox.
The fate that awaits you inside a black hole remains one of the greatest enigmas in modern physics, with theories still struggling to provide clarity on what happens to you as you meet your final end.
Understanding Accretion Disks
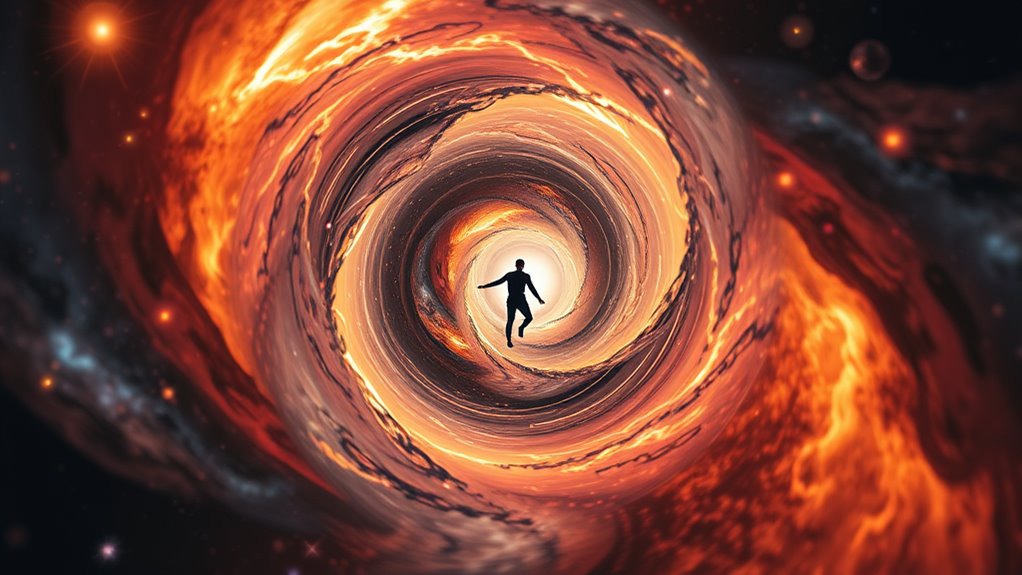
After exploring the fate inside a black hole, it's fascinating to shift focus to the phenomena surrounding these cosmic giants, particularly the formation of accretion disks. These disks emerge when material spirals towards a massive gravitational force, like a black hole or a star. As this material falls in, its small rotations amplify, causing it to form a disk structure instead of just falling straight in.
- A swirling mass of gas and dust, glowing with heat
- Tidal forces flattening the material into a thin plane
- Collisions generating X-rays that light up the cosmos
- Turbulent flows creating a dance of energy and momentum
The disk dynamics are essential here; as particles collide, they convert kinetic energy into heat, increasing the temperature of the disk. The balance between gravitational forces and heat pressure maintains the disk's shape, which can be thin or thick, depending on the conditions.
When pressure forces are minimal, the disk stays geometrically thin. However, if pressure is significant, it can puff up into a toroidal shape.
Material interactions within the disk are critical for accretion to occur. Turbulence and viscosity dissipate angular momentum, enabling the material to flow inward efficiently. This process releases energy more effectively than thermonuclear reactions, making accretion disks fundamental for powering energetic phenomena like quasars and X-ray binaries.
Observing these disks allows astronomers to infer the presence and mass of black holes, even when the black holes themselves remain hidden from view.
Radiation Emission From Jets
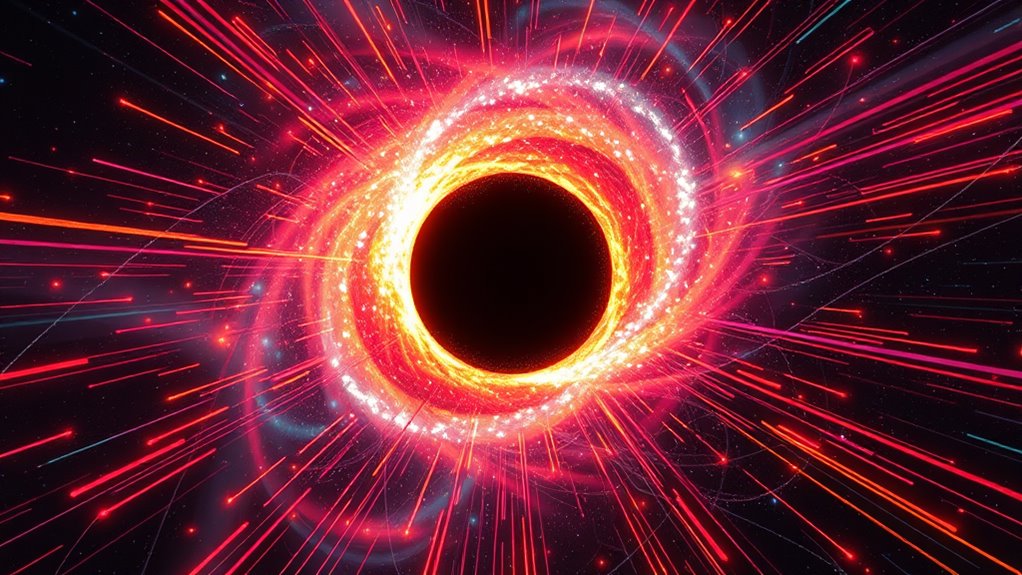
Black holes aren't just cosmic vacuum cleaners; they can also be powerful engines that emit jets of radiation. This emission arises from complex jet dynamics fueled by the black hole's rotation and its magnetic field. As the black hole spins, it twists magnetic field lines into a helix along its rotational axis, creating a voltage that extracts energy. This energy powers the jets, facilitating particle acceleration at various points along their length.
The magnetic interactions involved are essential for jet formation. Strong, orderly magnetic fields generate a current of electrons and positrons, which flow away from the black hole. The energy within these magnetic fields dissipates and transforms into ordinary radiation through radiation mechanisms like pair creation and synchrotron effects.
Observations, including those from the Event Horizon Telescope, provide evidence supporting the Blandford-Znajek process, which explains how these jets form and emit energy.
X-ray emissions from black hole jets further illustrate the role of particle acceleration. These emissions vary on short timescales, suggesting that the acceleration mechanisms are distributed throughout the jet, rather than concentrated solely at the black hole's center.
The variability in X-ray production indicates the involvement of high-energy electrons, complicating existing theories and prompting further research. Simulations tracking plasma particles help us understand the radiation spectra emitted by these jets, shedding light on the intricate interplay between magnetic fields and particle dynamics around black holes.
The Nature of the Singularity
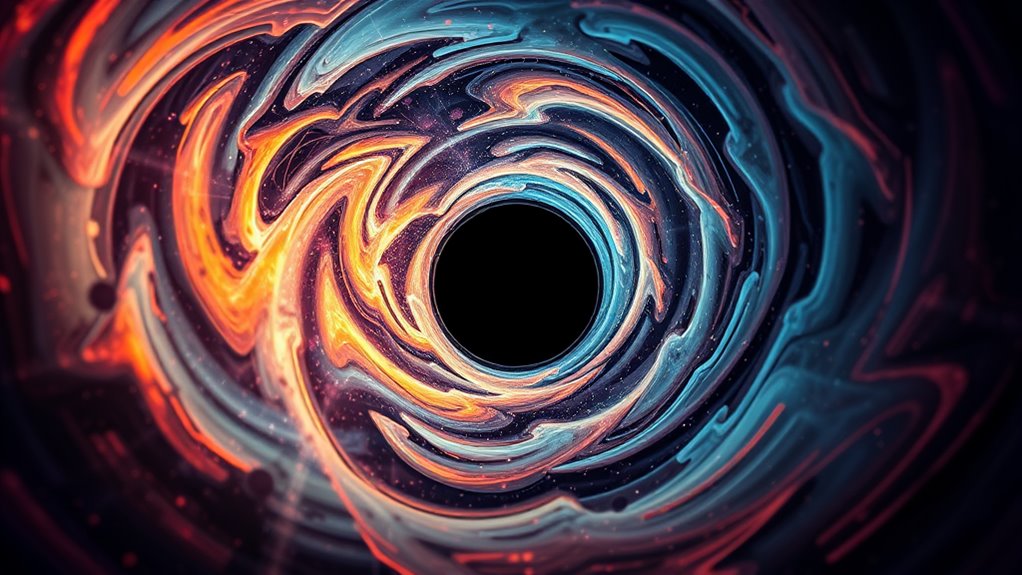
Understanding black holes goes beyond their powerful jets; it leads us to the enigmatic singularity at their core. This singularity is a region where the laws of physics as we comprehend them break down. Here, matter is crushed to infinite density, and the very fabric of spacetime behaves in bizarre ways.
You might picture:
- A point where everything you've ever known dissolves into nothingness.
- A swirling vortex, distorting time and space, pulling everything into its depths.
- An invisible barrier surrounding a hidden world of extreme gravity, where light can't escape.
- A chaotic dance of particles, pushed to their limits by unimaginable forces.
Singularities can take on various forms, known as singularity types. They can be timelike, allowing particles to move forward in time, or spacelike, where movement is stifled. Despite theories suggesting the existence of exotic matter that could prevent singularities, evidence remains elusive.
Infalling matter inevitably spirals into the singularity, facing extreme tidal forces that tear it apart.
Moreover, as you approach, the distinction between time and space blurs, making the journey feel surreal. Each black hole harbors its own unique singularity, whether it's a ring-shaped form in rotating black holes or the more commonly theorized central singularity.
In essence, the singularity represents not just a point of no return, but a profound mystery that challenges our comprehension of the universe itself.
Quantum Effects Near Singularity
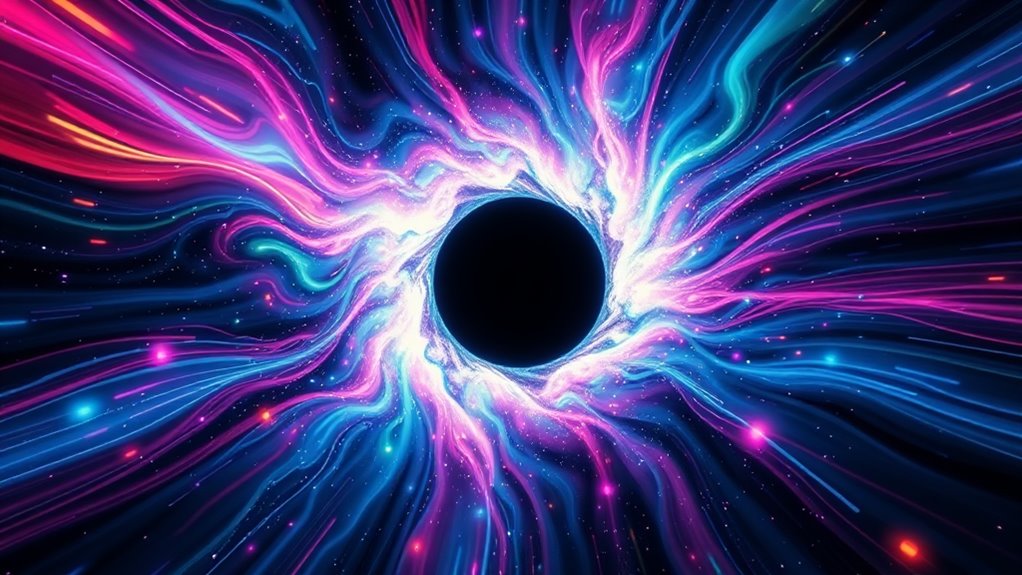
As you venture closer to a black hole's singularity, quantum effects begin to dominate, reshaping our understanding of reality itself.
You'll encounter the Unruh effect, which prevents a size-less singularity from forming by ensuring that energy conservation laws are upheld. Instead, the collapsing matter creates a finite singularity sphere, with its radius related to the square root of its mass. This phenomenon fundamentally alters the spacetime curvature around you, bending the very fabric of reality.
As you approach the inner horizon, you'll notice that gravitational effects intensify. Here, quantum effects emerge to block causality violations caused by diverging energy from Hawking radiation. The inner horizon acts like a barrier, splitting radiation into various levels, hinting at a chaotic interplay between quantum mechanics and general relativity.
Decoherence becomes apparent as you drift closer to the singularity. Quantum superpositions of particles outside the black hole lose their coherence, a process driven not by the matter present but by the geometry of spacetime itself.
This effect suggests a unique relationship between gravity, measurement, and quantum mechanics, revealing that even far-off particles feel the influence of the black hole's environment.
At this point, the exact behavior of matter and energy remains elusive without a complete theory of quantum gravity. However, it's clear that quantum entanglement and other phenomena play an essential role in shaping your experience as you approach this enigmatic region, marking the end of spacetime as you know it.
Long-Term Fate of Matter
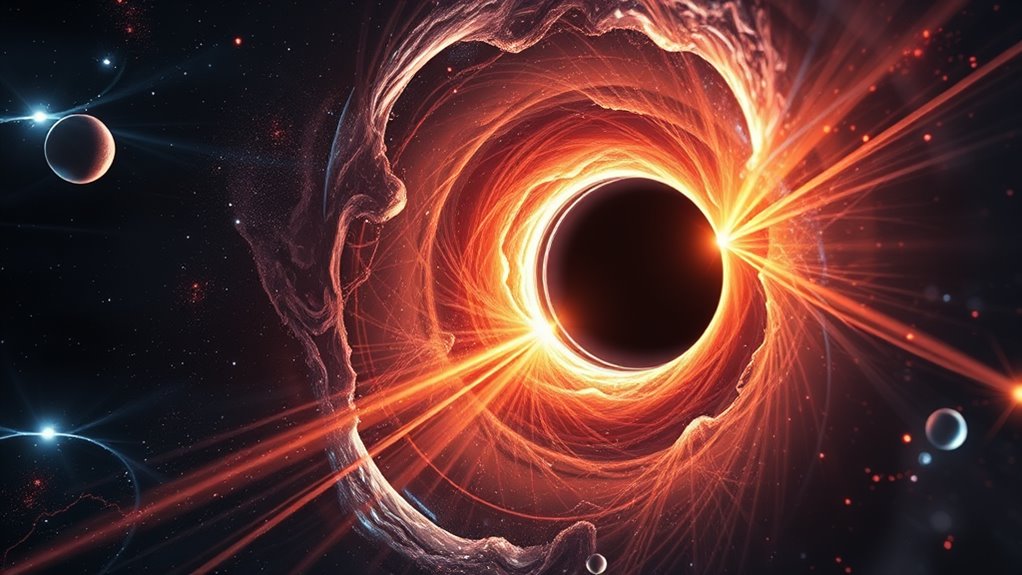
While you might think of black holes as cosmic vacuum cleaners, their long-term fate reveals a much more complex narrative about the destiny of matter in the universe. These enigmatic giants consume everything in their path—clouds of gas, entire stars, and even other black holes. Over billions of years, their insatiable appetite leads to the growth of supermassive black holes at the centers of galaxies, effectively resetting their cosmic clocks.
- Cosmic monsters growing larger with each meal
- Matter consumption that stretches over hundreds of billions of years
- The slow, inevitable process of black hole evaporation
- A universe where all matter might eventually collapse into one entity
As they consume matter, black holes delay their eventual evaporation, a phenomenon known as Hawking radiation. This process allows them to return energy to the universe, but the larger they are, the longer they take to disappear.
Eventually, they'll shrink and explode in a final burst of energy, though this event will be minuscule compared to other cosmic happenings.
In a universe where black holes reign supreme, the eventual fate of all physical matter could be catastrophic. It may be crushed into fundamental constituents, leaving behind a void that could lead to a new cycle of existence.
Frequently Asked Questions
Can I Survive Falling Into a Black Hole?
You can't survive falling into a black hole.
As you approach, you'd experience spaghettification effects, where extreme gravitational forces stretch your body into a long, thin shape.
Crossing the event horizon means you're trapped, unable to escape the black hole's grip.
The physics involved guarantees your disintegration before reaching the singularity.
Ultimately, no technology currently allows for survival in such conditions, making it a fatal experience every time.
What Happens to Light Near a Black Hole?
When you think about light near a black hole, it's fascinating how light bending occurs.
As light approaches the event horizon, its path curves dramatically due to the black hole's intense gravitational field. This bending can create mesmerizing effects, like rings of light from photons orbiting the black hole.
You'll notice that light shifts in color, appearing redder as it loses energy, revealing the profound influence black holes have on light and our universe.
Are Black Holes Portals to Other Universes?
You might wonder if black holes are portals to other universes. Various black hole theories suggest intriguing multiverse implications, like S-Brane shifts and wormholes connecting different points in space-time.
These theories propose that black holes could even allow travel to new universes. However, without empirical evidence, this remains speculative.
How Do Scientists Detect Black Holes?
Imagine you're a modern-day astronomer, peering through a telescope like it's the 1800s.
To detect black holes, you'd observe the effects of their gravity on nearby stars and gas. You'd look for X-rays emitted from hot gas spiraling towards the event horizon.
Additionally, you'd listen for gravitational waves created by merging black holes, revealing their presence.
Can Anything Escape a Black Hole Besides Radiation?
While nothing can escape a black hole once it crosses the event horizon, some phenomena can occur nearby.
Gravitational waves may ripple through space-time, and you might witness time dilation effects as you approach it.
Tidal forces can stretch objects, while cosmic strings could theoretically interact with the black hole's environment.
However, these events don't allow for escape; they simply highlight the extraordinary dynamics surrounding these mysterious entities.